RNA Therapeutics: The Future is Bright
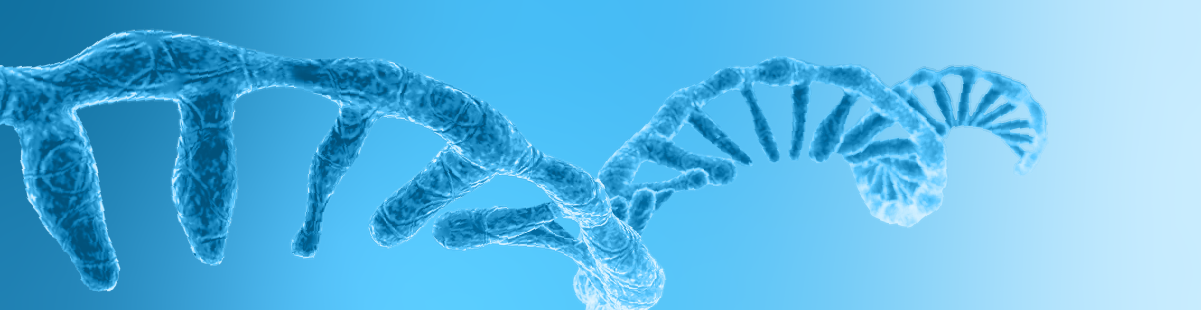
RNA therapeutics are a rapidly expanding category of drugs based on RNA molecules aimed at treating or preventing diseases. Recent clinical success and primarily the breakthrough of a series of fast-tracked and effective messenger RNA (mRNA)-based vaccines helping to conquer the COVID-19 pandemic (Kim, 2022; Zhu et al., 2022) did not only make “RNA” a household name but also reignited and propelled research interest in RNA therapeutics.
In this blog article, we will discuss the advantages of RNA therapeutics, concepts, and modes of action of different classes of RNA-based therapeutics. We will also give a glimpse into the RNA therapeutics currently being evaluated in clinical trials or having received approval.
How to drug the “undruggable”
Most conventional therapeutics currently on the market are based on small molecules that target the active sites of proteins implicated in the disease to inhibit or modify their function (Damase et al., 2021) or recombinant proteins that aim to bind to target proteins, replace unfunctional ones or supplement inadequate amounts of the target protein (Zogg et al., 2022). Currently, only 0.05 % of the human genome is targeted by protein-targeted therapeutics, small molecule chemicals, and antibodies (Zhu et al., 2022), as using small molecules and proteins as drugs have its limitations. Firstly, data show that only 10-14 % of all proteins have specific clefts and pockets suitable for binding small molecules (Hopkins and Groom, 2002; Dixon and Stockwell, 2009). Secondly, only a tiny fraction, as little as 1.5 % of the human genome, encodes proteins (Ezkurdia et al., 2014). Most of the genome is transcribed into noncoding RNAs (ncRNAs), which have also been implied as causative agents of many human diseases (Falese et al., 2021). As a result of all these limitations, many proteins, transcripts, and genes are “undruggable”, and hence a plethora of diseases are currently untreatable using conventional therapeutics.
Compared to conventional drugs, RNA-based therapeutics are promising to target previously untreatable, “undruggable” diseases thanks to their specific properties (Damase et al., 2021; Zhu et al., 2022). For example, RNA therapeutics can target mRNAs or noncoding RNAs via base pairing. Like this, they can target any interest gene by pairing it to the correct nucleotide sequence on the target RNA (Zhu et al., 2022). In addition, in vitro transcribed mRNAs can be applied for protein replacement treatment or immunization after entering cytoplasm (as in the case of COVID-19 mRNA vaccines).
Classes of RNA therapeutics
There are five different categories of RNA therapeutics as of now (Damase et al., 2021; Zogg et al., 2022): RNA aptamers, antisense oligonucleotides (ASOs), small interfering RNAs (siRNAs), microRNAs (miRNAs) and messenger RNAs (mRNAs) (Damase et al., 2021; Kim, 2022; Zhu et al., 2022; Zogg et al., 2022).
RNA aptamers
RNA aptamers are short single-stranded RNAs with well-defined secondary and tertiary structures which bind to various targets, such as proteins, peptides, carbohydrates, and other molecules. They are selected via SELEX (Systematic Evolution of Ligands by EXponential Enrichment) (Tuerk and Gold, 1990). They can serve as antagonists, agonists, and even carriers for other drugs (Adachi and Nakamura, 2019). An example of an aptamer is pegaptanib, the first-ever FDA-approved aptamer currently used to treat age-related macular degeneration (Ricci et al., 2020; Zogg et al., 2022). In this disease, the macula, a part of the retina that controls sharp vision, is damaged, causing blurred vision and vision loss (Ricci et al., 2020). Increased amounts of vascular endothelial growth factor (VEGF) were associated with the condition, and pegaptanib aptamer was found to have a high affinity for VEGF, causing it to be sequestered away from the VEGF receptor on target cells (Zhu et al., 2022).
Antisense oligonucleotides
ASOs are 15-25 nucleotides-long single-stranded RNAs, DNAs, or RNA-DNA heteroduplexes that can either promote or repress the expression of their complementary targets (Evers et al., 2015; Zhu et al., 2022). There are two classes of ASOs, dependent on RNase H (or ribozymes) (so-called occupancy-mediated degradation) (Wu et al., 2004) and independent from RNase H (an occupancy-only mechanism) (Baker et al., 1997). RNase H is an enzyme that degrades RNA that is part of the RNA-DNA heteroduplex, so in this case, once the ASOs bind to their target mRNA, RNase H is recruited to the complex and degrades the target mRNA (Zhu et al., 2022; Zogg et al., 2022). ASOs that utilize an occupancy-only mechanism are typically composed only of RNA and act as a steric block, thereby altering the gene expression of target transcripts using various mechanisms. For example, they can promote alternative splicing, cause nonsense-mediated mRNA decay (NMD), inhibit or activate translation, or block the interaction between miRNAs and their target mRNAs (Damase et al., 2021; Zhu et al., 2022; Zogg et al., 2022).
Three ASO-based drugs have so far received FDA approval: nusinersen, etepliersen, and inotersen (Damase et al., 2021; Feng et al., 2021; Zhu et al., 2022; Zogg et al., 2022). Nusinersen is approved for treating of spinal muscular atrophy (SMA), a potentially deadly disease causing weakness and atrophy of skeletal and respiratory muscles. SMA is caused by mutations in the SMN1 gene, resulting in the absence of SMN protein. SMN2, a homolog gene, is spliced such that there is exon skipping, resulting in a small amount of the SMN protein, which is insufficient for rescue. Nusinersen modifies the splicing of SMN2, enhancing the production of full-length, more stable SMN protein, significantly improving motor function in patients with SMA (Neil and Bisaccia, 2019). Etepliersen is used in the treatment of Duchenne muscular dystrophy, and, similar to nusinersen, is designed to modify the splicing of the pre-mRNA of the dystrophin gene, restoring the reading frame and leading to the production of the functional protein (Lim et al., 2017). Inotersen developed for treating familial amyloid polyneuropathy caused by the mutation in the transthyretin (TTR) gene, acts using a different mechanism. The expression of the mutated TTR gene renders an abnormal protein causing the disease. To fight the TTP buildup in organs, inotersen was designed to hybridize with the 3’ UTR of the TTP transcript, preventing its translation (Mahfouz et al., 2020).
siRNAs
RNA interference (RNAi) is a conserved biological response to double-stranded (ds)-RNA that mediates resistance to both endogenous parasitic and exogenous pathogenic nucleic acids and regulates the expression of protein-coding genes. siRNAs are double-stranded RNAs of 21-25 nucleotides (Zogg et al., 2022). They use RNAi pathway to modulate the expression of their target RNAs (Zhu et al., 2022). In native RNAi, endogenous small RNAs form a complex with Argonaute (AGO) protein to produce an RNA-induced silencing complex (RISC), which then suppresses the expression of their target mRNAs via sequence-specific binding (Kim, 2022). siRNA-based drugs use this same machinery. siRNA duplexes join the native RNAi pathway following their incorporation into RISC, where only the guide strand is retained by the AGO protein while the passenger strand is discarded, and the guide strand then focuses RISC activity against its target transcript (Kim, 2022; Zhu et al., 2022; Zogg et al., 2022).
Currently, there are four siRNA therapeutics on the market: patisiran used to treat above mentioned amyloid polyneuropathy, givosiran that is used in treatment against acute hepatic porphyria, lumasiran for the treatment of primary hyperoxaluria and inclisiran, approved in 2021 to treat atherosclerotic cardiovascular disease, by lowering LDL cholesterol levels (Kim, 2022; Zogg et al., 2022).
miRNAs
miRNAs are naturally occurring, small, non-coding RNA molecules that regulate the expression of a whole array of mRNAs by blocking translation or promoting degradation of their target transcripts. Their extraordinary targeting capability makes them an excellent therapeutic potential. With them being naturally occurring molecules endogenous to our cells, there is also less chance for immunogenic response with miRNA therapeutics than their other synthetic counterparts (Zogg et al., 2022). The miRNA-based therapeutics could be categorized into two types: miRNA mimics and miRNAs inhibitors. Mimics are dsRNA molecules that mimic miRNAs, identical in sequence to the endogenous miRNA duplexes, which lead to the repression of target mRNAs and reduction in protein levels. On the other hand, miRNA inhibitors are single-stranded RNA oligos designed to interfere with miRNAs, thereby restoring protein synthesis. While no miRNAs are FDA-approved therapeutics, many are in clinical development (Zhu et al., 2022; Zogg et al., 2022).
mRNAs
mRNAs are a great candidate for treating the disease with a known genetic component. They are based on the fact that even exogenous mRNAs, delivered into the cell via, e.g., lipid nanoparticles , are translated into functional proteins. mRNAs can be used as a replacement or supplementation therapy – where mRNA compensates for a defective gene/protein, or to supply therapeutic proteins, such as in the case of enzyme replacement (Kim, 2022).
mRNAs encoding antigens and/or adjuvants can be used as vaccines to elicit protective immunity against infectious diseases or to harness the immune system to fight cancer as therapeutic vaccines. The COVID-19 pandemic has showcased the advantages of RNA technology for vaccination, as out of all COVID-19 vaccines under development, the first two to have received emergency use authorization by the FDA were RNA-based (Damase et al., 2021; Kim, 2022; Zhu et al., 2022; Zogg et al., 2022). Because COVID-19 caused a pandemic over a very short period, an effective vaccine needed to be developed as soon as possible, and mRNA vaccines have the advantage of a relatively short development process. In addition, since mRNA were studied as vaccine candidates for so long, the existing knowledge was so vast that it was easily utilized quickly (Kim, 2022).
mRNA vaccines can also be used as personalized medicines for targeting specific tumors. First, a unique tumor mutation is identified and then included in an mRNA transcript. This exogenous transcript will then express this mutation, inducing a specific immune response targeting cells expressing these antigens, allowing the targeted destruction of these cells within the body (Kim, 2022).
At the moment, only two mRNA-based vaccines are FDA-approved (both for COVID-19), and two more are in clinical trials, another for COVID-19 in Phase III, and one for rabies prevention in Phase I. Several mRNA therapeutics are in clinical trials, including those against cystic fibrosis and advanced melanoma (Zogg et al., 2022).
Summary and Future Perspective
RNA therapeutics are multifunctional and very versatile, promising to treat and prevent many, so far, untreatable human diseases, using different mechanisms. They are additionally cheaper, easier, and faster to develop than conventional therapeutics. Several current challenges for RNA therapeutics, such as cell-specific delivery, are yet to be solved, to release their full potential. Given the attention there are currently receiving, interdisciplinary approaches and developments in modern science, we will positively see many more approved RNA therapeutics in the upcoming years.
When developing therapeutics of any kind, it is essential to perform all the tests and confirmations of activity, safety, and efficiency with well-established methods and products. If you would like to learn about the importance and benefit of RNA-Seq in the discovery process and in assessing the activity and effectiveness of RNA-based therapeutics, check out the following blog article.
References: